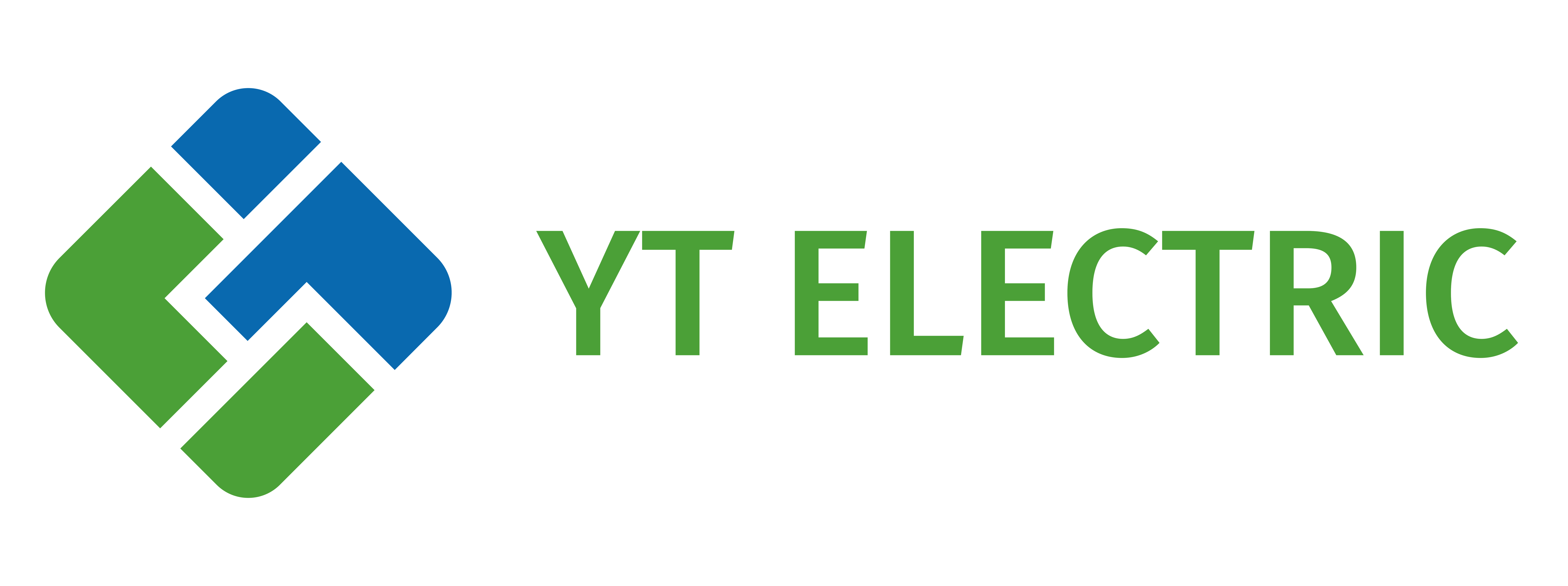
Before diving into the architecture and types of battery energy storage systems (BESS), it is essential to familiarize ourselves with key terminology commonly used in this field. Various parameters are crucial in describing the behavior and performance of BESS.
· Capacity [Ah]: This represents the amount of electric charge the system can supply to a connected load while maintaining an acceptable voltage level. The capacity is influenced by the battery technology and is typically defined at specific temperatures and discharge currents.
· Nominal Energy [Wh]: This is the total energy a battery can deliver from a fully charged state to complete discharge. It is calculated by multiplying the capacity by the battery voltage. Since it is dependent on capacity, it is also affected by temperature and current.
· Power [W]: Defining the output power of a BESS is complex as it depends on the connected load. However, the nominal power indicates the output during typical discharge conditions.
· Specific Energy [Wh/kg]: This parameter describes the energy a battery can store in relation to its mass.
· C Rate: This denotes the rate of charge and discharge relative to the battery’s maximum capacity. At 1C, the battery discharges fully in one hour.
· Cycle: A full charge-discharge-charge sequence. There is no universal standard defining what constitutes a cycle.
· Cycle Life: The number of cycles a battery can complete before its performance degrades significantly.
· Depth of Discharge (DoD): Represents the percentage of the battery discharged. A 100% DoD indicates full discharge.
· State-of-Charge (SoC, %): Indicates the current charge level of a battery.
· Coulombic Efficiency: This reflects the efficiency of charge transfer within the battery. It is the ratio of the charge (Ah) discharged to the charge required to return to the initial state. Most batteries, except for lead-acid batteries, exhibit near-perfect Coulombic efficiency.
Main Types of Electrochemical Energy Storage Systems
Various battery technologies rely on different chemical elements and reactions. Today, lead-acid and lithium-ion batteries are the most common, though nickel-based, sulfur-based, and flow batteries have also played significant roles in the industry. Below is a brief overview of the main advantages of commonly used battery technologies.
Lead-Acid Batteries
These are widely used in everyday applications. They consist of a negative lead electrode, a positive electrode of lead dioxide, and an electrolyte solution of sulfuric acid.
Advantages: Low cost and technological maturity.
Disadvantages: See Table 1 for details.
Nickel-Cadmium (Ni-Cd) Batteries
These batteries dominated portable systems for years before lithium-ion technology emerged. They offer strong power performance and rapid recharging.
Advantages and Disadvantages: Refer to Table 2.
Nickel-metal-hydride (NiMH) batteries, an evolution of Ni-Cd, provide approximately 40% higher specific energy.
Lithium-Ion (Li-Ion) Batteries
Lithium-ion batteries, with lithium metal as the anode, deliver exceptional specific energy. However, they face challenges like dendrite formation on the anode, which can cause short circuits and overheating.
Advantages and Disadvantages: Refer to Table 3.
Composition of a BESS
A BESS consists of multiple interconnected components, each with specific roles and control requirements. Below is a summary of these main components:
1. Battery System: Comprising several battery packs, interconnected to achieve the desired voltage and current levels.
2. Battery Management System (BMS): Ensures safe operation by maintaining voltage, current, and temperature levels within limits. It also balances the state of charge across cells.
3. Power Conversion System (PCS): Converts DC power from the battery to AC for external use. PCS units often include monitoring and auxiliary services for optimal operation.
4. Energy Management System (EMS): Monitors and manages energy flow based on application requirements. EMS typically integrates with a SCADA (Supervisory Control and Data Acquisition) system for overall system monitoring and control.
5. Transformers: Depending on the system size, medium-voltage/low-voltage or high-voltage/medium-voltage transformers are used for grid connection.
Integrating PV Modules with BESS
As renewable energy becomes integral to future electrical systems, integrating a BESS with renewable sources, such as PV modules, enhances both the energy system and the power plant.
Benefits of PV and BESS Integration:
· Mitigating Generation Volatility: A BESS smooths fluctuations in power output caused by clouds or sudden peaks, creating a more stable generation curve (Figure 4).
· Peak Shaving: Reduces peak generation, resulting in a smoother curve.
· Grid Support: Provides ancillary services such as voltage control, frequency regulation, and reactive power compensation, enabling better grid integration.
Coupling Options for PV Modules and BESS:
1. DC Coupling: The PV and BESS are connected on the DC side, sharing inverters and AC components. Common in residential and small-scale plants, but requires advanced control logic for DC voltage and battery management.
2. AC Coupling After the Inverter: PV and BESS each have dedicated inverters, making this suitable for residential and large-scale plants. This approach avoids the complexity of DC coupling.
3. AC Coupling at the Point of Connection (POC): PV and BESS systems share only the interconnection facility, with independent sections for each system.
Subscribe to us to enjoy event prices and get some of the best prices.